Therapeutic oligos: promising types and their applications
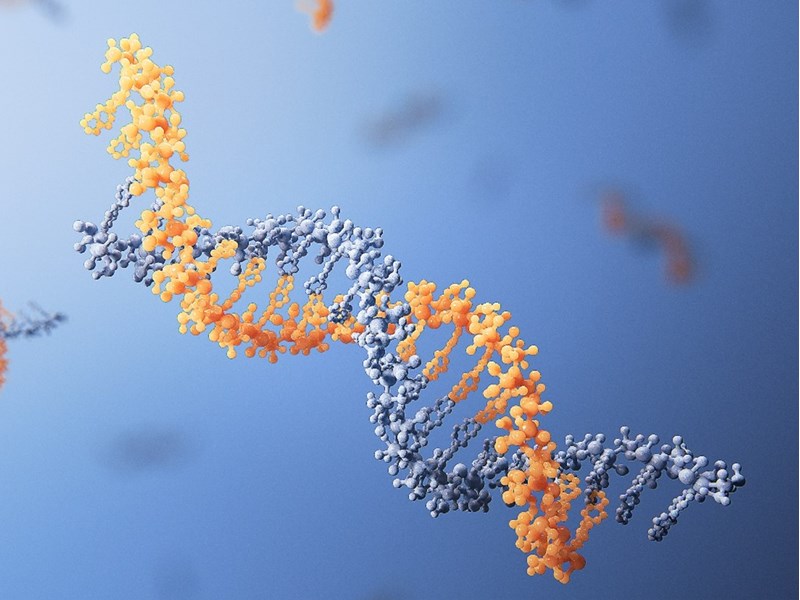
This article will explore the mechanisms behind some of the most promising types of therapeutic oligonucleotides and their diverse applications in treating various medical conditions.
Therapeutic oligonucleotides are quickly gaining recognition as a potent class of medications that can address numerous diseases. These short nucleic acid strands can precisely modulate gene expression and protein activity, making them highly adaptable tools for modern medicine.
1. ASOs
Antisense oligonucleotides (ASOs) are synthetic, single-stranded nucleic acids designed to bind to specific RNA sequences and regulate gene expression. They work by targeting RNAs and using various methods to modify gene expression. One key mode of actions involves recruiting RNase H, an enzyme that degrades the targeted double stranded RNA, reducing its levels and preventing translation of undesirable protein1. ASOs can also block ribosomes from binding to mRNA to prevent protein synthesis or alter RNA splicing patterns to promote specific transcript isoforms. In some cases, they can enhance protein production by blocking inhibitory elements such as miRNAs, negative regulatory elements, and more.2
ASOs have a wide range of therapeutic uses. They can silence or modify the expression of defective genes in genetic disorders3, target and suppress oncogenes or restore tumor suppressor genes in cancer4 and inhibit viral replication in viral infections5. This versatility makes ASOs an exciting and powerful tool in modern medicine, offering precise and effective ways to manage gene expression across various diseases.
2. Aptamers
Aptamers are short, synthetic nucleic acid molecules that fold into unique three-dimensional shapes, enabling them to bind tightly and selectively to target molecules like proteins, small molecules, or even entire cells6. This precise binding can block interactions with other cellular components or induce changes that impair the target's activity.
Aptamers have a broad range of therapeutic applications due to their versatility and precision. In cancer treatment, aptamers can deliver therapeutic agents directly to tumor cells, reducing side effects by minimizing impact on healthy tissues7. They are also being explored for infectious diseases, where they can bind to viral proteins or host cell receptors to prevent viral entry and replication8. Additionally, aptamers show promise in treating autoimmune disorders by selectively modulating immune responses9. Their chemical synthesis and modification provide significant advantages in terms of stability, scalability, and reduced immunogenicity compared to traditional antibodies, making them a powerful tool in modern therapeutic strategies.
3. siRNA
Small interfering RNAs (siRNAs) operate by degrading targeted mRNA sequences to silence specific genes. The process begins when double-stranded siRNA enters the cell. Inside the cell, the RNA-induced silencing complex (RISC) unwinds the siRNA strands (2). The antisense strand then guides RISC to the complementary mRNA target (3). Upon binding, the RISC complex cleaves the mRNA, leading to its degradation by the cellular machinery and thereby preventing protein synthesis (4)10.
Figure 1: siRNAs way of action
The precision of siRNAs allows them to target only specific mRNA, making them suitable for treating diseases such as certain hereditary disorders and some cancers. This specificity minimizes off-target effects but requires careful design to avoid unintended gene silencing and immune responses 11.
siRNA can downregulate almost all genes and therefore, they can be used for numerous types of diseases such as cancer and genetic disorders12. For example, siRNA can target and silence oncogenes, slowing down or stopping cancer proliferation13. For genetic disorders, it can silence mutant genes, offering potential treatments14. Moreover, siRNAs can be used to target viral RNA thus preventing viruses from replicating in host cells15.
4. miRNA
MicroRNAs (miRNAs) are small, non-coding RNA molecules essential for gene regulation. Unlike siRNAs, miRNAs can modulate multiple mRNAs through partial complementarity. This ability allows them to regulate a network of genes effectively. While both miRNAs and siRNAs form a duplex with the RISC complex, the first ones typically bind at the 3′ UTR of target mRNAs. This binding can block ribosome assembly or promote mRNA degradation16.
miRNAs are crucial in various cellular processes, including cell development, differentiation, proliferation, and apoptosis. In diagnostics, they can serve as biomarkers for the early detection and monitoring of different kinds of diseases such as Alzheimer’s, cardiovascular diseases, and more.
Not only do they have significant diagnostic potential, but their ability to regulate gene expression simultaneously across different pathways makes them an interesting therapeutic technology. miRNAs regulation can help mitigate many different types of diseases, such as cancer, cardiovascular, and neurodegenerative conditions17,18. This can be achieved thanks to two different miRNA technologies: miRNA mimics and antimiRs. miRNA mimics can restore suppressed miRNA levels, while antimiRs can inhibit overactive miRNA function. By regulating gene expression, they mitigate disease progression.
5. CpG oligodeoxynucleotides
CpG oligodeoxynucleotides (CpG ODNs) are synthetic single-stranded DNA molecules. They activate the immune system through the recognition of unmethylated cytosine-phosphate-guanine (CpG) motifs derived from bacterial DNA. These CpG motifs are specifically bond by Toll-like receptor 9 on immune cells. When bound to these receptors, this process triggers a signaling cascade leading to the production of cytokines19.
In medical treatments, CpG ODNs are used to boost the immune response against cancers and infectious diseases. They can be administered alone or in combination with other therapies, such as chemotherapy and vaccines20. For instance, in cancer therapy, CpG ODNs can be injected directly into tumors or given systemically to enhance the body's natural ability to fight cancer cells21. Clinical studies have shown that CpG ODNs can significantly improve the effectiveness of existing treatments, leading to better outcomes for patients.
Conclusion
Therapeutic oligonucleotides enclose a wide range of different technologies, each with unique mechanisms and applications. While this article provides a brief overview of some key approaches, it's important to acknowledge the existence of other significant technologies such as guide RNAs and more. These advances illustrate the diverse and expanding potential of oligonucleotide-based therapies in modern medicine. The field is rapidly evolving, promising innovative treatments and breakthroughs soon.
Do you want to start your therapeutic oligos journey with us? More info here
References
- Kole, R., Krainer, A. R. & Altman, S. RNA therapeutics: beyond RNA interference and antisense oligonucleotides. Nat. Rev. Drug Discov. 11, 125–140 (2012).
- Lauffer, M. C., van Roon-Mom, W., Aartsma-Rus, A., & N = 1 Collaborative. Possibilities and limitations of antisense oligonucleotide therapies for the treatment of monogenic disorders. Commun. Med. 4, 6 (2024).
- Hill, S. F. & Meisler, M. H. Antisense Oligonucleotide Therapy for Neurodevelopmental Disorders. Dev. Neurosci. 43, 247–252 (2021).
- Quemener, A. M. et al. The powerful world of antisense oligonucleotides: From bench to bedside. WIREs RNA 11, e1594 (2020).
- Tarn, W.-Y., Cheng, Y., Ko, S.-H. & Huang, L.-M. Antisense Oligonucleotide-Based Therapy of Viral Infections. Pharmaceutics 13, 2015 (2021).
- Nimjee, S. M., White, R. R., Becker, R. C. & Sullenger, B. A. Aptamers as Therapeutics. Annu. Rev. Pharmacol. Toxicol. 57, 61–79 (2017).
- Morita, Y., Leslie, M., Kameyama, H., Volk, D. & Tanaka, T. Aptamer Therapeutics in Cancer: Current and Future. Cancers 10, 80 (2018).
- Chen, J., Zhou, J., Peng, Y., Xie, Y. & Xiao, Y. Aptamers: A prospective tool for infectious diseases diagnosis. J. Clin. Lab. Anal. 36, e24725 (2022).
- Yasmeen, F., Seo, H., Javaid, N., Kim, M. S. & Choi, S. Therapeutic Interventions into Innate Immune Diseases by Means of Aptamers. Pharmaceutics 12, 955 (2020).
- Friedrich, M. & Aigner, A. Therapeutic siRNA: State-of-the-Art and Future Perspectives. BioDrugs 36, 549–571 (2022).
- Hu, B. et al. Therapeutic siRNA: state of the art. Signal Transduct. Target. Ther. 5, 101 (2020).
- Lam, J. K. W., Chow, M. Y. T., Zhang, Y. & Leung, S. W. S. siRNA Versus miRNA as Therapeutics for Gene Silencing. Mol. Ther. - Nucleic Acids 4, e252 (2015).
- Mahmoodi Chalbatani, G. et al. Small interfering RNAs (siRNAs) in cancer therapy: a nano-based approach. Int. J. Nanomedicine Volume 14, 3111–3128 (2019).
- Zhang, M. M., Bahal, R., Rasmussen, T. P., Manautou, J. E. & Zhong, X. The growth of siRNA-based therapeutics: Updated clinical studies. Biochem. Pharmacol. 189, 114432 (2021).
- Kang, H. et al. Small interfering RNA (siRNA)-based therapeutic applications against viruses: principles, potential, and challenges. J. Biomed. Sci. 30, 88 (2023).
- Davidson, B. L. & McCray, P. B. Current prospects for RNA interference-based therapies. Nat. Rev. Genet. 12, 329–340 (2011).
- Zhou, S. et al. miRNAS in cardiovascular diseases: potential biomarkers, therapeutic targets and challenges. Acta Pharmacol. Sin. 39, 1073–1084 (2018).
- Forterre, A., Komuro, H., Aminova, S. & Harada, M. A Comprehensive Review of Cancer MicroRNA Therapeutic Delivery Strategies. Cancers 12, 1852 (2020).
- Zhang, Z. et al. CpG Oligodeoxynucleotides for Anticancer Monotherapy from Preclinical Stages to Clinical Trials. Pharmaceutics 14, 73 (2021).
- Dongye, Z., Li, J. & Wu, Y. Toll-like receptor 9 agonists and combination therapies: strategies to modulate the tumour immune microenvironment for systemic anti-tumour immunity. Br. J. Cancer 127, 1584–1594 (2022).
- Hanagata, N. CpG oligodeoxynucleotide nanomedicines for the prophylaxis or treatment of cancers, infectious diseases, and allergies. Int. J. Nanomedicine Volume 12, 515–531 (2017).