Evolution of Multiplex PCR: Benefits, Challenges & Key Applications
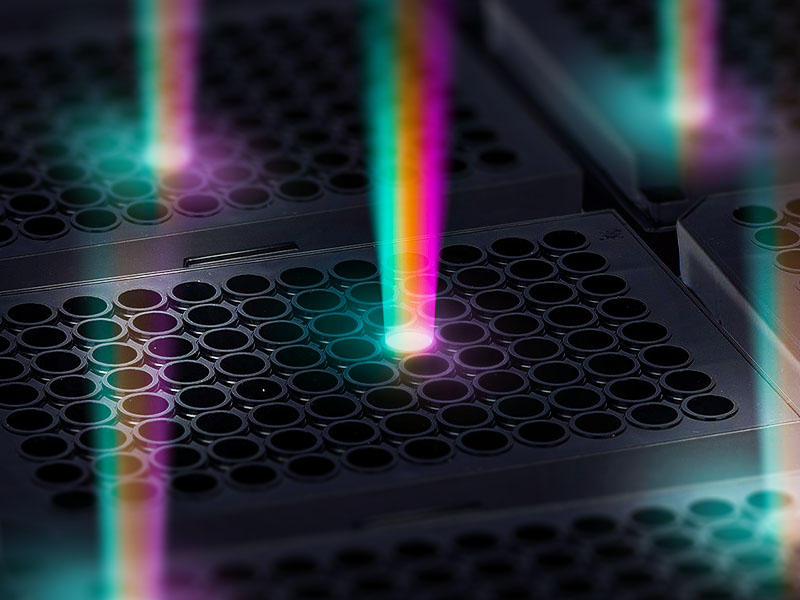
Multiplex PCR is a powerful technique that enables the simultaneous detection of multiple genetic targets in a single reaction. Widely used in diagnostics, research, and quality control, it maximizes efficiency while conserving valuable samples. Discover multiplex PCR evolution & key applications.
What is multiplex PCR?
Multiplex PCR allows multiple DNA or RNA targets to be amplified simultaneously within a single reaction, ranging from simpler formats like duplex PCR (amplifying two targets) to highly complex assays targeting numerous sequences at once. With standard PCR, multiple primer pairs share a common reagent mix—including DNA polymerase, dNTPs, buffer, and magnesium ions—and amplicons are distinguished by size. The introduction of fluorescent reporters, such as labeled primers or TaqMan® probes, has revolutionized multiplex PCR by allowing the detection of same-size amplicons. These reporters provide real-time, quantitative data, eliminating the need for post-PCR processing and significantly enhancing accuracy and workflow efficiency. Advanced fluorescence-based technologies in some digital PCR platforms further expand multiplexing capabilities, enabling the detection of numerous targets in a single reaction.
What are the advantages of multiplex PCR?
Multiplex PCR delivers significant benefits, making it indispensable for labs worldwide:
- Sample Preservation: Ideal for scenarios where samples are scarce or valuable, enabling maximal information retrieval from limited material.
- Increased Throughput: After setup, multiplex PCR speeds up time-to-result by detecting multiple targets in a single reaction, requiring less run and pipetting steps and leading to quicker data generation.
- Cost Savings: Once optimized, a single reagent mix supports multiple targets, reducing costs associated with reagents and consumables.
- Data Reliability: Multiplex assays enable the inclusion of co-detectable controls, such as Sample Processing Controls (SPC) and Internal Positive Controls (IPC). These TaqMan®-based controls ensure the accuracy and correct interpretation of data.
What are the challenges of multiplex PCR?
Multiplex PCR offers many advantages, but its initial setup can be challenging due to several factors:
- Complex Assay Development: Designing specific, non-reactive primers for multiple targets and optimizing reaction conditions (e.g., annealing temperature, cycling profile) require significant effort. Multiple targets competing for shared reagents can lead to imbalanced amplification and reduced sensitivity without careful optimization.
- Handling: Managing multiple primer and probe pairs increases the risk of pipetting errors and contamination during reaction setup, potentially compromising assay accuracy. These issues can be mitigated by using pre-mixed reagents and robust protocols.
- Detection Challenges: Multiplex assays require advanced fluorescence detection systems and non-overlapping fluorophores to accurately distinguish multiple signals, adding to the complexity of assay design.
- Initial Time and Cost: Developing and validating a multiplex PCR assay involves greater time and resource investment compared to singleplex assays. However, these upfront efforts yield significant time and cost savings once the assay is optimized and routinely implemented.
Who benefits most from multiplex PCR?
While setting up a multiplex PCR assay can be challenging, it is especially valuable for scientists who handle high volumes of precious samples and routinely test for the co-occurrence of multiple targets within the same sample, such as:
- Researchers and Clinicians working with thousands of limited or precious samples to detect specific genes expression.
- Lab technicians conducting repetitive measurements on a multitude of similar samples, such as in routine diagnostic assays or quality controls.
- Scientists working in high-throughput facilities requiring rapid processing of numerous samples in parallel.
Multiplex PCRs: A natural evolution with PCR techniques
Multiplex PCR evolved alongside PCR techniques, benefiting from advancements in fluorophore technologies, thermocyclers, software tools, or microfluidics. Over time, it has expanded to include multiple variants, each tailored to specific applications. Here are the key milestones in its evolution, shedding light on how each step has contributed to its current capabilities and applications 1,2.
Figure 1. Key milestones of multiplex PCR evolution. Inspired from 1,2,3.
Multiplex end-point PCR: Gel electrophoresis
Multiplex endpoint PCR amplifies multiple DNA regions in a single reaction, distinguishing the resulting products by size through gel electrophoresis. This technique uses specific primers for each target sequence, with amplified fragments visualized under UV light after electrophoresis.
One of the earliest applications was the detection of genetic deletions in the Duchenne muscular dystrophy (DMD) gene, as demonstrated by Chamberlain et al. in 1988 4.
This groundbreaking work showcased the potential of multiplex PCR for the simultaneous analysis of multiple regions of a single gene.
Multiplex end-point PCR: Capillary electrophoresis and fluorophores
Capillary electrophoresis (CE) and fluorophores have revolutionized multiplex PCR by significantly enhancing its resolution and multiplexing capacity. While CE enables the separation of amplicons by size with high precision, the use of distinct fluorophores attached to primers allows the differentiation of amplicons based on fluorescence emission. The combination of size and fluorescence has dramatically expanded multiplexing capabilities, enabling the simultaneous detection of up to 20 or more targets.
One prominent application is the analysis of Short Tandem Repeats (STRs), which can be of identical size but differ in sequence. This technique is particularly valuable in forensic applications and paternity testing, where genetic identification of individuals relies on unique STR profiles 5. Modern systems can amplify and detect up to 26 loci simultaneously, making it a powerful tool for genetic analysis.
A variation of PCR that leverages CE and fluorescent primers is nested multiplex PCR, which enhances sensitivity and specificity by performing two sequential rounds of PCR reactions. The first round can amplify broader target regions, while the second uses internal primers to refine detection.
An example of nested multiplex PCR in action is the CoVarScan approach for detecting SARS-CoV-2 variants 6. In this study, an initial multiplex PCR was performed to amplify regions containing mutations characteristic of various variants, followed by a second round of PCR to increase specificity. This method enabled rapid and flexible detection of all SARS-CoV-2 variants of concern, showcasing the utility of nested multiplex PCR for pathogen detection and surveillance.
Multiplex Real Time qPCR with TaqMan® probes
The evolution from endpoint PCR to real-time quantitative PCR (qPCR) marked a significant advancement in molecular biology for nucleic acid analysis. TaqMan®-based multiplex qPCR uses primer pairs and sequence-specific probes to detect and quantify multiple DNA or RNA targets simultaneously. For RNA analysis, reverse transcription qPCR (RT-qPCR) adds an initial step where RNA is converted into complementary DNA (cDNA) using reverse transcriptase, enabling RNA targets such as viral genomes or mRNA to be analyzed with the same precision as DNA. Each probe in qPCR is double-labeled with a fluorescent reporter and a quencher, which suppresses fluorescence when the probe is intact. During amplification, DNA polymerase cleaves the probe, separating the reporter from the quencher and releasing fluorescence. This signal increases proportionally to the amount of amplified target, allowing precise quantification. Distinct fluorescent dyes for each probe enable the simultaneous detection of multiple targets in a single reaction, making qPCR and RT-qPCR indispensable tools for both DNA and RNA analysis.
Multiplex qPCR is cost-effective, fast, and ideal for high-throughput workflows, cementing its role as the gold standard for routine diagnostics. A prominent example was the widespread use of RT-qPCR during the SARS-CoV-2 pandemic, which not only enabled massive testing but is now applied to differentiate between SARS-CoV-2, Influenza A/B 7 and other respiratory pathogens. In routine testing, multiplex panels allow simultaneous detection of respiratory or gastrointestinal pathogens, including bacteria, viruses, and parasites, as well as bloodstream pathogens for sepsis diagnostics and various resistance genes 8. Beyond clinical applications, real-time PCR is extensively used in food safety to detect foodborne pathogens such as Salmonella, Listeria, and E. Coli 9, as well as genetically modified organisms (GMOs) in food products 10. Additionally, it plays a critical role in monitoring microbial contamination in water and soil samples 11.
Loop-Mediated Isothermal Amplification (LAMP)
Loop-Mediated Isothermal Amplification (LAMP) is a rapid DNA amplification technique performed at a constant temperature, eliminating the need for thermocyclers. Using 4 to 6 primers targeting 6 to 8 DNA regions, LAMP delivers high sensitivity and speed. It can sometimes bypass DNA extraction, directly amplifying nucleic acids from crude samples like saliva or blood, making it ideal for point-of-care testing.
LAMP offers versatile detection options, including fluorescent reporting for real-time monitoring, colorimetric detection for simple visual readouts, portable dipstick lateral flow assays, and turbidity-based methods. While traditionally used as a qualitative method, LAMP can achieve semi-quantitative results by monitoring fluorescence intensity over time, but it lacks the precise quantification capabilities of techniques like qPCR.
Multiplexing LAMP adds complexity, as primer interactions and competition can reduce efficiency and accuracy. With multiple targets, meticulous optimization is required to maintain specificity and prevent false-positive or false-negative results 12.
Nevertheless, an interesting study demonstrated the potential of multiplex LAMP by combining two singleplex assays into a duplex system for SARS-CoV-2 detection. Amplicon-specific probes were developed to link the products of both reactions, ensuring that only connected LAMP products generated a signal 13. This duplex LAMP, paired with a lateral flow readout, achieved sensitive and accurate detection, highlighting its suitability for field diagnostics with minimal equipment.
Multiplex Ligation-Dependent Probe Amplification (MLPA)
Also based on capillary electrophoresis and fluorescent primers, other innovations have emerged to further enhance target detection and quantification. One such advancement is Multiplex Ligation-Dependent Probe Amplification (MLPA).
MLPA relies on probe pairs hybridizing to adjacent regions of a target sequence. After hybridization, the probes are ligated to form a single contiguous molecule, which is subsequently amplified using a single pair of universal primers in a PCR reaction. The amplification products are then analyzed by capillary electrophoresis, where their size and quantity correspond to specific targets and their relative abundance.
A notable application of MLPA is methylation-specific MLPA (MS-MLPA), widely used for diagnosing Beckwith-Wiedemann Syndrome (BWS)14. MS-MLPA is one of the most robust diagnostic tools for BWS, detecting the three most common molecular alterations: IC1 gain of methylation (GOM), IC2 loss of methylation (LOM), and paternal uniparental disomy (patUPD), as well as genomic copy number variations (CNVs). Hence, MS-MLPA assay can detect both copy number variations and methylation defects of the 11p15.5 critical region within one single experiment, providing a comprehensive and reliable diagnostic method for clinical laboratories.
High Resolution Melting (HRM)
High-Resolution Melting (HRM) analysis, combined with qPCR and non-specific intercalating dyes like SYBR® Green, is a powerful technique for detecting DNA sequence variations through unique melting profiles. Unlike traditional qPCR, which quantifies nucleic acids, HRM analyzes the melting behavior of double-stranded DNA as it separates into single strands. This enables the differentiation of amplicons with subtle sequence differences, such as mutations, SNPs, or epigenetic modifications. SYBR® Green binds to double-stranded DNA and emits fluorescence proportional to the DNA quantity, but its non-specific binding can complicate multiplexing. HRM overcomes this challenge by leveraging unique melting temperatures (Tm) to distinguish between amplicons. With careful primer design, the combination of SYBR® Green and HRM provides a cost-effective and efficient solution for specific multiplex qPCR applications.
SYBR® Green-based multiplex qPCR is primarily used in research and development or proof-of-concept studies. In resource-limited settings, it may serve as a cost-effective preliminary diagnostic tool for simple targets, particularly when probe-based methods are unavailable. As an example, multiplex SYBR® Green real-time qPCR enabled the detection of antimicrobial resistance genes (pbp2b, ermB, mef) in Streptococcus pneumoniae directly from cerebrospinal fluid. Using melting temperature (Tm) analysis, the method differentiated amplicons, enabling rapid identification of resistance profiles, streamlining treatment decisions for bacterial meningitis 15.
Multiplex digital PCR
Digital PCR (dPCR) is a highly precise technique for detecting and quantifying DNA or RNA targets. Like quantitative PCR (qPCR), it relies on sequence-specific probes, such as TaqMan® probes, for target detection. However, while qPCR monitors fluorescence in real time during amplification cycles, dPCR measures fluorescence at the end-point. This is achieved by partitioning the sample into thousands of individual micro-compartments, enabling absolute quantification using Poisson statistics and eliminating the need for standard curves required in qPCR. However, compared to qPCR, dPCR comes with higher costs per sample and lower throughput, which can limit its use in routine, large-scale applications 16.
The multiplexing capacity of digital PCR (dPCR) primarily depends on the number of detection channels available on the instrument, but it is also enhanced by technological advancements such as color combinationa, fluorescence amplitudeb, and partitioning systems 17,18.
Emulsion-based systems partition samples into thousands of microdroplets. While earlier systems supported moderate multiplexing (detecting 2 to 6 targets), advancements have improved this capacity. However, challenges such as spectral overlap among fluorophores within droplets still necessitate careful assay design and optimization to ensure accurate detection.
Microfluidics-based systems utilize fixed microchambers for sample partitioning, physically separating reactions and enhancing signal resolution. With fluorescence amplitude-based multiplexing, dPCR platforms utilizing microfluidic nanoplates and six-color detection systems can simultaneously detect up to 12 targets in a single reaction while minimizing interference.
Crystal Digital PCR™ employs an innovative hybrid partitioning method that combines emulsion-based droplet partitioning with the spatial organization of microfluidic chambers, ensuring high sensitivity and precise quantification. In its 7-color detection version, Crystal Digital PCR™ platforms maximize multiplexing capacity by integrating color combination and fluorescence amplitude-based multiplexing, allowing the simultaneous detection of up to 21 targets in a single reaction, making it a powerful tool for high-complexity applications.
a Color combination multiplexing uses distinct fluorophores or color blends (e.g., red, green, blue, cyan, magenta, yellow) to differentiate targets, maximizing the platform's multicolor detection capacity.
b Fluorescence amplitude-based multiplexing distinguishes multiple targets within the same fluorescence channel by differentiating them based on fluorescence intensity. This is achieved by using dual-labeled TaqMan® probes with the same dye and quencher but at varying concentrations, ensuring distinct fluorescence intensities for each target after end-point amplification.
The Table 1 compiles some key applications of multiplex digital PCR.
Table 1. Multiplexed digital PCR key applications.
Fields | Applications | References |
Cancer diagnostics |
Highly sensitive detection of rare mutations (e.g., KRAS, NRAS and BRAF SNPs)in liquid biopsies. Detection of multiple mutations in cfDNA EGFR in patient plasmas. Detection of PIK3CA mutations in the plasma of metastatic breast cancer patients. |
19
20
21 |
Clinical biomarkers diagnostics |
miRNA panel detection & quantification. |
22 |
Infectious disease testing |
Simultaneous detection and viral DNA load quantification of different Human papillomavirus Types. Detection of Co-infection with Human Herpesviruses 6A and 6B (HHV-6A and HHV-6B) in Clinical Samples. Clinical validation of multiplex dPCR for diagnostic of bloodstream infections in ICU practise. |
23
24
25 |
Prenatal and Genetic Testing |
Supports non-invasive prenatal testing (NIPT) by detecting chromosomal abnormalities (e.g., Down syndrome) and rare genetic mutations with absolute quantification. |
26 |
Food safety and Environmental Testing |
Simultaneous absolute quantification of 3 bacteria in aquaculture. Detection of SARS-CoV-2 Variants of Concern in wastewater. |
27
28 |
Multiplex PCR in NGS workflow
Multiplex PCR is not just a standalone endpoint technique but a pivotal component of next-generation sequencing (NGS) workflows. One of its advanced implementations, anchored multiplex PCR for targeted next-generation sequencing, has revolutionized targeted sequencing by enabling the enrichment of rare or low-abundance targets 29. This approach amplifies regions surrounding structural variations, such as insertions, deletions, and rearrangements, without requiring prior knowledge of breakpoints. Anchored multiplex PCR facilitates compatibility with amplicon-based sequencing methods, ensuring efficient library preparation and high coverage of target regions 30. These capabilities make multiplex PCR indispensable for applications like cancer mutation profiling, pathogen detection, and inherited disease analysis in NGS workflows.
Conclusions
Multiplex PCR has become a cornerstone in molecular biology high-throughput workflows by conserving valuable samples and reducing time, costs, and resources. Its ability to simultaneously amplify and detect multiple targets in a single reaction has made it indispensable for applications such as pathogen detection, rare mutation analysis in oncology, prenatal testing or pharmacogenomics.
Ongoing advancements in reagents, fluorescent dyes, and detection systems are expanding the horizons of multiplex PCR, enabling it to tackle even more complex challenges. Its integration into next-generation sequencing workflows and the development of ultra-multiplex platforms highlight its evolving role in driving breakthroughs in research, diagnostics, and quality control. As these innovations continue, multiplex PCR will remain an essential tool in addressing the growing demands of modern molecular biology and personalized medicine.
Your multiplex solutions with Eurogentec
With decades of experience, Eurogentec is a trusted partner dedicated to helping scientists overcome the challenges of multiplex PCR while simplifying every step of the workflow.
Eurogentec offers comprehensive support for multiplex assay development, including advanced primer design assistance together with a wide selection of Access™ dyes and quenchers for your qPCR probes or other modified oligonucleotides. All primers and TaqMan®probes for qPCR or dPCR are available in a range of quality grades, from research to diagnostic-grade, tailored to meet your specific needs. Moreover, we offer cross-contamination free NGS oligo for all your NGS applications.
To enhance the reliability of multiplex assays, Eurogentec provides multiplex-compatible Internal Positive Controls (IPCs) and Sample Processing Controls (SPCs), ensuring accurate results and robust workflow validation.
To further optimize multiplex performance, Eurogentec developed the Takyon® Ultra, a reverse transcription qPCR MasterMix designed with increased multiplex capabilities for the sensitive and specific detection of RNA targets, including SARS-CoV-2, Influenza, and RSV.
Your multiplex assay is ready?
Eurogentec’s cleanroom assembly service can prepare pre-mixed reagents to minimize pipetting errors and improve reproducibility. Save time now.
Loved exploring multiplex PCR?
Read our last blog article about ATTO dyes and discover why they are the perfect match for quantitative and digital PCR probes—delivering precision, brightness, and flexibility for complex assays.
References
- Zhu, H. et al. PCR Past, Present and Future. BioTechniques 69, 317–325 (2020).
- De La Soldedad Lagunes-Castro, M., López-Monteon, A., Guzmán-Gómez, D. & Ramos-Ligonio, A. Metabarcoding and Digital PCR (dPCR): Application in the Study of Neglected Tropical Diseases. in New Advances in Neglected Tropical Diseases (ed. Aparecida Sperança, M.) (IntechOpen, 2023). doi:10.5772/intechopen.106272.
- Pomari, E., Piubelli, C., Perandin, F. & Bisoffi, Z. Digital PCR: a new technology for diagnosis of parasitic infections. Microbiol. Infect. 25, 1510–1516 (2019).
- Chamberlain, J. S., Gibbs, R. A., Rainer, J. E., Nguyen, P. N. & Thomas, C. Deletion screening of the Duchenne muscular dystrophy locus via multiplex DNA amplification. Nucleic Acids Res. 16, 11141–11156 (1988).
- Cornelis, S. et al. Multiplex STR amplification sensitivity in a silicon microchip. Rep. 8, 9853 (2018).
- Clark, A. E. et al. Multiplex Fragment Analysis for Flexible Detection of All SARS-CoV-2 Variants of Concern. Chem. 68, 1042–1052 (2022).
- Shu, B. et al. Multiplex Real-Time Reverse Transcription PCR for Influenza A Virus, Influenza B Virus, and Severe Acute Respiratory Syndrome Coronavirus 2. Infect. Dis. 27, 1821–1830 (2021).
- Gray, J. & Coupland, L. J. The increasing application of multiplex nucleic acid detection tests to the diagnosis of syndromic infections. Infect. 142, 1–11 (2014).
- Kawasaki, S. et al. Evaluation of a multiplex PCR system for simultaneous detection of Salmonella spp., Listeria monocytogenes, and Escherichia coli O157:H7 in foods and in food subjected to freezing. Foodborne Pathog Dis.6(1):81-9 (2009).
- Datukishvili, N., Kutateladze, T., Gabriadze, I., Bitskinashvili, K. & Vishnepolsky, B. New multiplex PCR methods for rapid screening of genetically modified organisms in foods. Microbiol. 6, (2015).
- Sidrach-Cardona R. et al., Prevalence of antibiotic-resistant fecal bacteria in a river impacted by both an antibiotic production plant and urban treated discharges. Science of The Total Environment 1, 220-227 (2014).
- Crego-Vicente, B., Del Olmo, M. D., Muro, A. & Fernández-Soto, P. Multiplexing LAMP Assays: A Methodological Review and Diagnostic Application. J. Mol. Sci. 25, 6374 (2024).
- Bhadra S. et al. High-Surety Isothermal Amplification and Detection of SARS-CoV-2. mSphere 6, e00911-20(2021).
- Priolo, M. et al. MS-MLPA is a specific and sensitive technique for detecting all chromosome 11p15.5 imprinting defects of BWS and SRS in a single-tube experiment. J. Hum. Genet. 16, 565–571 (2008).
- Souza, M. B. et al. Multiplex real-time PCR using SYBR Green: Unspecific intercalating dye to detect antimicrobial resistance genes of Streptococcus pneumoniae in cerebrospinal fluid. PLOS ONE 17, e0269895 (2022).
- Nazir, S. Medical diagnostic value of digital PCR (dPCR): A systematic review. Eng. Adv. 6, 100092 (2023).
- Madic, J. et al. Three-color crystal digital PCR. Detect. Quantif. 10, 34–46 (2016).
- Whale, A.S. et al. Fundamentals of multiplexing with digital PCR. Biomolecular Detection and Quantification. 10:15–23 (2016).
- Neugebauer, M. et al. Generic Reporter Sets for Colorimetric Multiplex dPCR Demonstrated with 6-Plex SNP Quantification Panels. J. Mol. Sci. 25, 8968 (2024).
- Ntzifa, A., Kotsakis, A., Georgoulias, V. & Lianidou, E. Detection of EGFR Mutations in Plasma cfDNA and Paired CTCs of NSCLC Patients before and after Osimertinib Therapy Using Crystal Digital PCR. Cancers 13, 2736 (2021).
- Corné, J. et al. Development of multiplex digital PCR assays for the detection of PIK3CA mutations in the plasma of metastatic breast cancer patients. Rep. 11, 17316 (2021).
- Busato, F., Ursuegui, S., Deleuze, J.-F. & Tost, J. Multiplex digital PCR for the simultaneous quantification of a miRNA panel. Chim. Acta 1335, 343440 (2025).
- Rotondo, J. C. et al. Simultaneous Detection and Viral DNA Load Quantification of Different Human Papillomavirus Types in Clinical Specimens by the High Analytical Droplet Digital PCR Method. Microbiol. 11, 591452 (2020).
- Velluci, A. et al., Using Droplet Digital PCR to Detect Coinfection of Human Herpesviruses 6A and 6B (HHV-6A and HHV-6B) in Clinical Samples Methods Mol Biol, 1768:99-109 (2018).
- Wu, J. et al. Clinical validation of a multiplex droplet digital PCR for diagnosing suspected bloodstream infections in ICU practice: a promising diagnostic tool. Care 26, 243 (2022).
- Li, Y., Tan, G. & Zhou, Y. Digital PCR and its applications in noninvasive prenatal testing. Funct. Genomics 21, 376–386 (2022).
- Netzer, R., Ribičić, D., Aas, M., Cavé, L. & Dhawan, T. Absolute quantification of priority bacteria in aquaculture using digital PCR. Microbiol. Methods 183, 106171 (2021).
- Boogaerts, T. et al. Optimization and Application of a Multiplex Digital PCR Assay for the Detection of SARS-CoV-2 Variants of Concern in Belgian Influent Wastewater. Viruses 14, 610 (2022).
- Zheng, Z. et al. Anchored multiplex PCR for targeted next-generation sequencing. Med. 20, 1479–1484 (2014).
- Xie, N. G. et al. Designing highly multiplex PCR primer sets with Simulated Annealing Design using Dimer Likelihood Estimation (SADDLE). Commun. 13, 1881 (2022).